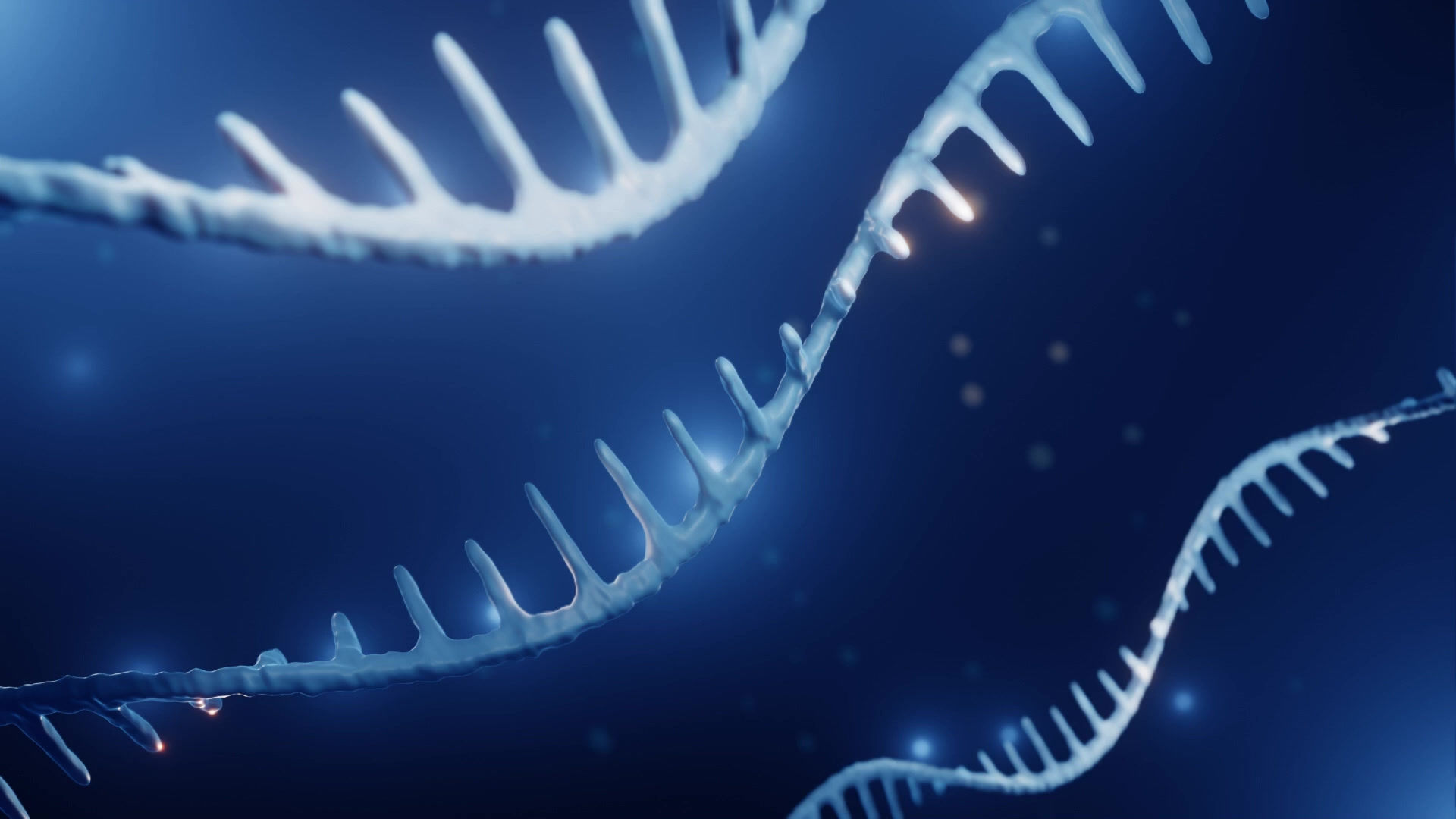
Deciphering mRNA modifications
on a transcriptome-wide scale
The Rentmeister group focuses on chemical modifications of mRNA, a key aspect of epitranscriptomics. Our research aims to elucidate the functions of modified DNA and RNA bases to decrypt the chemical information level of nucleic acids beyond sequence information. We have developed innovative approaches such as MePMe-seq and CAPturAM, which enable precise mapping and identification of RNA modifications and methyltransferase targets, advancing our understanding of the regulatory functions of RNA modifications in gene expression.
Internal modifications of mRNA have emerged as widespread and versatile regulatory mechanism to control gene expression at the post-transcriptional level (1). Here, at the Institute of Chemical Epigenetics – Munich (ICEM) of the LMU we aim to elucidate the functions of modified DNA and RNA bases in the cell to decrypt the chemical information level of the nucleic acids above the sequence information. The Rentmeister group focuses on chemical modifications of mRNA (epitranscriptomics).
Global view via metabolic labeling.
RNA modifications are more abundant and more diverse than DNA modifications. Most modifications are found in tRNA and rRNA, but even in mRNA, currently 10 different internal modifications have been described (1). Identifying the positions of mRNA modifications on a transcriptome-wide scale relies on the ability to make a modified nucleoside amenable to next generation sequencing (NGS). Most groups in the field take a biochemical approach and use antibodies. This requires a specific antibody for each type of modification. Since the chemical modifications are very small compared to antibodies, bias of antibodies is a known problem in the field. The Rentmeister group developed a chemical biology approach to make mRNA modifications amenable to next generation sequencing (3).
Most of the internal mRNA modifications are methylations involving the co-factor S-adenosyl-L-methionine (SAM), however, simultaneous detection of different methylation sites in the same sample has remained elusive. Our approach allows to assign different methylation sites in eukaryotic RNA with extremely high precision via next-generation sequencing (2, 3). It relies on metabolic labeling with propargyl-selenohomocysteine (PSH) in combination with click chemistry to detect N6-methyladenosine (m6A) and 5-methylcytidine (m5C) sites in mRNA with single nucleotide precision in the same sequencing run (MePMe-seq). Intracellular formation of the corresponding SAM analogue leads to detectable levels of N6-propargyl-A (prop6A) and 5-propargyl-C (prop5C). We have developed the mass spectrometric analysis for quantification of modified nucleosides from cell samples, which requires synthesis of the standards. MePMe-seq overcomes the problems of antibodies for enrichment and sequence-motifs for evaluation, limiting previous methodologies. MePMe-seq is the first method enabling simultaneous mapping of several types of RNA modifications (3). This technological advance will be important to study the interconnectivity of the different modifications and improve our understanding of mechanisms and functions of the RNA methylome.

Figure 1: Scheme of MePMe-seq (metabolic propargylation for methylation sequencing). a, Metabolic labeling of cells with propargyl-selenohomocysteine (PSH) leads to methionine adenosyl transferase (MAT)-catalyzed formation of SAM-analogue and propargylation of methyltransferase (MTase) target sites. b, After cell lysis, poly(A)+ RNA is isolated and fragmented. c, Propargylated fragments react with biotin azide in a copper-catalyzed azide-alkyne cycloaddition (CuAAC) and are bound to streptavidin-coated magnetic beads. d, On-bead reverse transcription (RT) terminates at modified sites. e, Libraries for NGS are prepared. Modified sites are detected as steep coverage drops.
b) Enzyme-specific view via chemo-enzymatic modification
Modified nucleotides impact all aspects of eukaryotic mRNAs and contribute to regulation of gene expression at the transcriptional and translational level. At the 5′ cap, adenosine as first transcribed nucleotide is often N6-methyl-2′-O-methyl adenosine (m6Am). This modification is tissue dependent and reversible, pointing to a regulatory function. CAPAM was recently identified as methyltransferase responsible for m6Am formation (4), however, the direct identification of its target transcripts proves difficult. Antibodies do not discriminate between internal N6-methyl adenosine (m6A) and m6Am. We have developed CAPturAM, an antibody-free chemical biology approach for direct enrichment and identification of physiological CAPAM-targets (5). We harness CAPAM’s co-substrate promiscuity to install propargyl groups on its targets. Subsequent functionalization with an affinity handle allows for their enrichment.
In a first proof-of-concept, we used wildtype and CAPAM–/– cells and successfully confirmed known CAPAM-targets by qRT-PCR. CAPturAM allows to identify the transcription start nucleotide (TSN) N6-methylation status of any transcript of interest. In future work at the LMU, we aim to integrate this approach into NGS sequencing to determine CAPAM-targets on a transcriptome-wide scale. The chemo-enzymatic modification of the 5′ cap is not limited to identifying targets of CAPAM. Our group has a long-standing experience in cap-modifying enzymes (6). In future work, we aim to use N2-modifying enzyme Giardia lamblia trimethylguanosine synthase (GlaTgs) to modify m7G-caps with clickable handles (7). This can be used to isolate mRNAs as a complementary approach to the typically performed binding of the poly(A) tail to oligo(dT) beads and/or to sequence these mRNAs. As the 5′ cap is installed very early during transcription, this technology bears potential to identify transcripts with 5′ cap but without poly(A) tail. This includes histone-mRNAs but also several long non-coding RNAs. It could also shed light on mRNAs with short poly(A) tails that are lost when purifying via oligo(dT) beads. As several processes, including early development in the zebrafish and local translation in synapses, are regulated via cytoplasmic polyadenylation, this technology may provide new insights into the transcriptome and regulatory mechanisms at the post-transcriptional level.
References
1. Zaccara, S.; Ries, R. J.; Jaffrey, S. R.
Reading, writing and erasing mRNA methylation.
Nature Reviews Molecular Cell Biology 2019, 20 (10), 608–624.
2. Hartstock, K.; Nilges, B. S.; Ovcharenko, A.; Cornelissen,
N. V.; Püllen, N.; Lawrence‐Dörner, A.; Leidel, S. A.; Rentmeister, A.
Enzymatic or in Vivo Installation of Propargyl Groups in Combination with Click Chemistry
for the Enrichment and Detection of Methyltransferase Target Sites in RNA.
Angewandte Chemie 2018, 57 (21), 6342–6346.
3. Hartstock, K.; Kueck, N. A.; Spacek, P.; Ovcharenko, A.; Hüwel, S.;
Cornelissen, N. V.; Bollu, A.; Dieterich, C.; Rentmeister, A.
MePMe-Seq: Antibody-Free Simultaneous M6A and M5C Mapping
in MRNA by Metabolic Propargyl Labeling and Sequencing.
Nature Communications 2023, 14 (1), 7154.
4. Akichika, S.; Hirano, S.; Shichino, Y.; Suzuki, T.; Nishimasu, H.; Ishitani, R.;
Sugita, A.; Hirose, Y.; Iwasaki, S.; Nureki, O.; Suzuki, T.
Cap-Specific Terminal N 6 -Methylation of RNA by an RNA Polymerase II–Associated Methyltransferase.
Science 2019, 363 (6423).
5. Muthmann, N.; Albers, M.; Rentmeister, A.
CAPturAM, a Chemo‐Enzymatic Strategy for Selective Enrichment
and Detection of Physiological CAPAM‐Targets.
Angewandte Chemie International Edition 2022, 62 (4).
6. Bollu, A.; Peters, A.; Rentmeister, A.
Chemo-Enzymatic Modification of the 5′ Cap to Study MRNAs.
Accounts of Chemical Research 2022, 55 (9), 1249–1261.
7. Schulz, D.; Josephin Marie Holstein; Rentmeister, A.
A Chemo‐Enzymatic Approach for Site‐Specific Modification of the RNA Cap.
Angewandte Chemie 2013, 52 (30), 7874–7878.